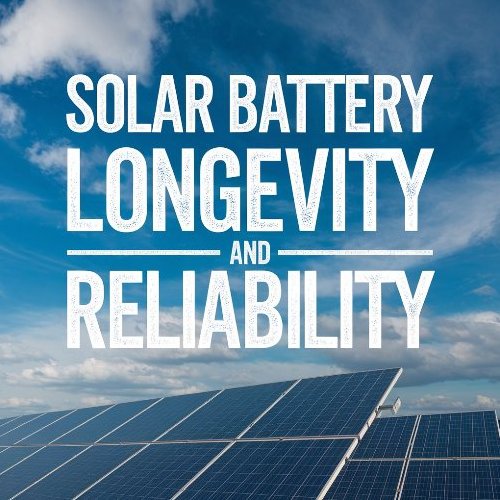
Batteries have become integral to modern solar energy systems mainly due to rising electric costs and changes in net metering policies. These batteries store excess energy generated during the day, ensuring backup power during outages and greater energy independence.
Two main types of solar batteries dominate the market: lead-acid and lithium-ion batteries. Each has unique advantages, costs, and lifespan considerations. This solar battery longevity case study examines how long solar LFP batteries last, the factors affecting their longevity, and tips for maximizing their lifespan.
Table of Contents
LFP Performance and Research Insights
Lithium-ion batteries, particularly those using lithium iron phosphate (LFP) chemistry, are the gold standard in solar energy storage. Although they are more expensive upfront than lead-acid options, their longevity and efficiency make them a more cost-effective choice over time.
The following graph shows various lithium-ion battery chemistries and their power degradation percentage over a number of cycles. A cycle is defined as a full charge and discharge of the battery. As this graph notes, LFP batteries retain their power capacity significantly better than other lithium-ion chemistries.
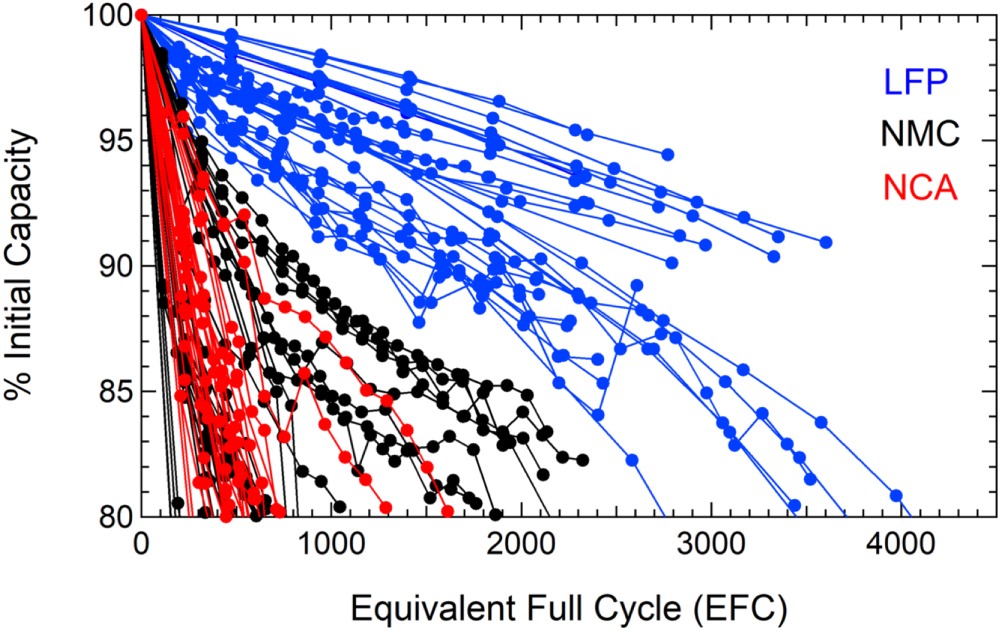
- Lifespan: On average, LFP batteries can last 15–20 years and endure 6,000 to 10,000 cycles before their capacity diminishes to 70-80%.
- Cost Comparison: Lithium-ion batteries may cost up to six times more than lead-acid batteries. However, their high energy density, longer lifespan, and reduced maintenance requirements balance the equation.
The Preger Study and Benefits of LFP
The Preger study, published in 2020 in the Journal of The Electrochemical Society, investigated the degradation of commercial lithium-ion batteries with LFP, NCA, and NMC chemistries under different cycling conditions. The researchers identified distinct degradation trends by varying temperature, depth of discharge (DOD), and discharge rate within manufacturer-specified limits. LFP cells exhibited the longest cycle life, underscoring their durability, while temperature effects varied:
To support future research, the study introduced batteryarchive.org, a public repository for battery cycling data that enables researchers to visualize, analyze, and compare datasets collaboratively. This initiative enhances understanding of battery degradation and improves lifetime prediction models. The findings highlight LFP’s robustness—making it a strong candidate for residential solar storage—and reveal the chemistry-specific nature of degradation, offering valuable insights for battery selection and system design.
Benefits of Lithium-Ion Batteries Found in the Study
- Energy Efficiency: Lithium-ion batteries have a higher round-trip efficiency, ensuring more energy storage and use per charge cycle.
- Durability: They can withstand deeper depths of discharge (up to 80%), enabling more usable capacity.
- Low Maintenance: Unlike lead-acid batteries, LFP batteries do not require regular watering or corrosion checks.
- Safety: LFP chemistry is more stable, reducing the risks of overheating or fire.
Durability of Solar Batteries: Insights from Real-World Data
Solar battery systems have proven durable, but like all technologies, they are not immune to occasional issues. Our monitoring data from thousands of solar homes with installed battery systems shows us insights into their performance and reliability over time.
Early Failures: The “Infant Mortality” Period
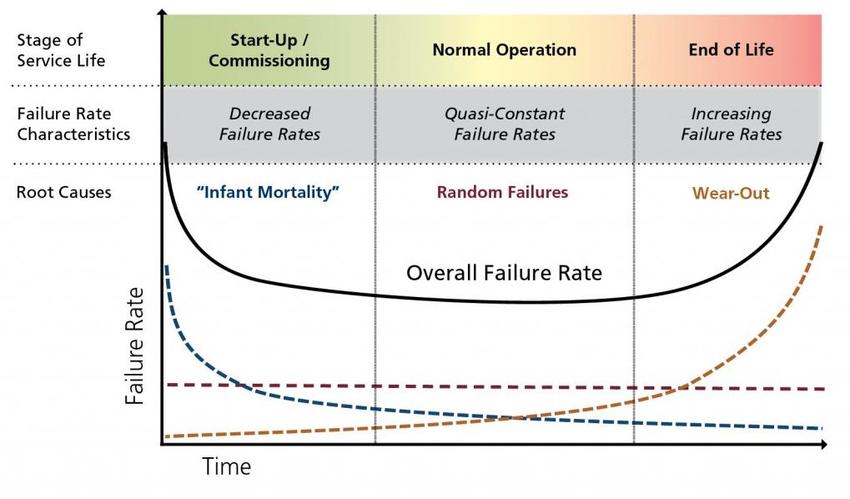
Solar battery systems, like many electronic components, follow the principles of the bathtub curve in reliability engineering. Approximately 1 in 100 systems experienced some component-related issue during the first two years after commissioning. These early failures, often termed infant mortality, are typically caused by:
- Manufacturing defects in batteries or their associated components.
- Installation errors including incorrect wiring or improper configuration of the battery management system (BMS).
- Integration issues with inverters, charge controllers, or other solar energy system components.
The good news is that most reputable manufacturers and installers address these early failures promptly under warranty.
Steady-State Reliability: Low Failure Rates After Stabilization
The failure rate drops significantly once solar battery systems pass the critical first 300 days of operation, marking the bathtub curve’s stabilization phase.
- Key Observations: During this phase, batteries operate with exceptional reliability, often exhibiting failure rates well below 1% per year.
- Factors Contributing to Durability:
- Advanced manufacturing techniques and stringent quality control during production.
- Improvements in Battery Management Systems (BMS) that monitor and optimize performance.
End-of-Life Wear-Out Failures
As solar batteries approach the end of their designed lifecycle, typically after 10-15 years or 6,000–10,000 cycles, failure rates rise. This wear-out phase is characterized by:
- Capacity degradation: Batteries lose the ability to hold their full charge, reducing usable energy storage.
- Increased internal resistance: This leads to inefficiencies and potential overheating.
- Higher likelihood of mechanical or chemical failures: Components experience fatigue after years of cycling.
Although failures at this stage are natural, proper planning and maintenance, such as proactively monitoring capacity and replacing components, can mitigate disruptions.
Key Technological Components of an LFP Battery System
Advanced technological components working in harmony bolster the longevity and reliability of LFP batteries. These elements, from the Battery Management System (BMS) to integrated inverters, ensure that LFP batteries deliver consistent power while maximizing lifespan. This section dissects the key technologies behind LFP systems, showing how they optimize performance, enhance safety, and adapt to solar demands.
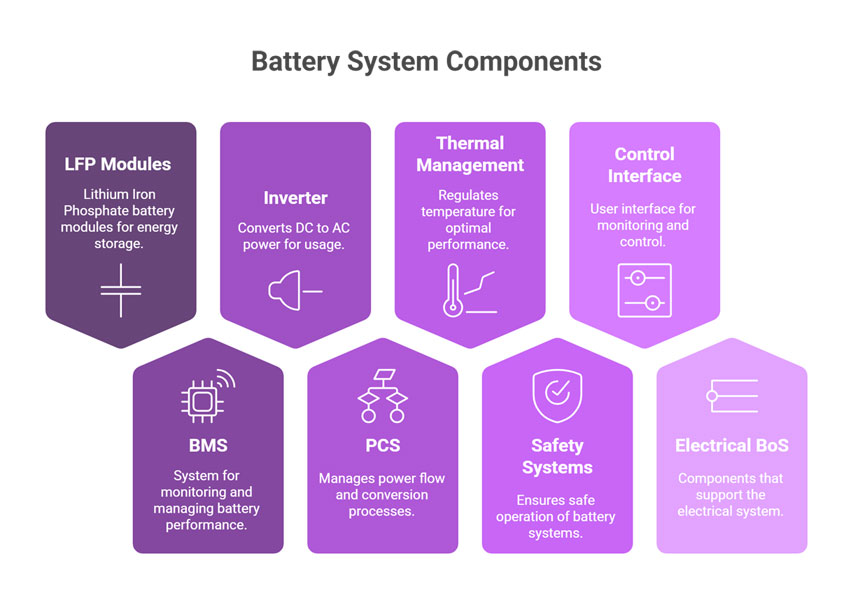
1. Battery Management System (BMS)
Battery Management System (BMS), which functions as a power distribution box and control center. The BMS ensures energy flow, monitors usage patterns, and integrates with third-party systems for enhanced versatility.
Key Functions of a Typical BMS:
- Partial and Whole-House Backup Power: Provides energy during outages, keeping critical appliances running. Some newer, sophisticated technologies can dynamically “shed” high consumption loads so as not to overload the system.
- Solar Self-Consumption: Optimizes the use of solar energy generated on-site by discharging stored energy when home consumption spikes above solar production.
- Load Shifting and Time-of-Use Control: Reduces electricity costs by shifting energy use to off-peak hours.
- Off-Grid Battery Backup Mode: Enables uninterrupted power in isolated systems.
- VPP (Virtual Power Plant) Support: Contributes to broader energy grids for load balancing and resource optimization.
- Generator Control: Manages external generators for supplemental power.
By integrating these capabilities, the BMS enhances energy efficiency and also adapts to diverse user needs.
2. Battery and Inverter Integration
Many LFP battery modules come alongside a bidirectional DC/AC inverter or an attached integrated inverter, delivering both energy storage and power conversion in a single enclosure. This compact and modular design simplifies installation and operation.
Key Specifications:
- Energy and Power Output: Total energy (kWh) is determined by battery specs, while a combination of the inverter and battery determines power output. For example, a battery could carry a 13.5 kWh capacity, but only be able to output 5 kW at one time.
- High Overload Capacity: Many LFP batteries and inverters can output up to 10 kW continuous power for 7-10 seconds to handle the inrush current of high-demand appliances.
- Parallel Operation: Supports scalability by linking multiple batteries together for larger energy needs.
- Integrated Protection Mechanisms: Features hardware and software safeguards against overvoltage, overcurrent, and other electrical anomalies.
This integration of inverter and battery technology makes the units space-efficient and reliable.
Core Technologies
Several key technologies underpin the advanced functionality of LFP systems:
- Power Conversion System (PCS): Enables seamless bidirectional energy conversion between DC (battery) and AC (household appliances).
- Battery Management System (BMS): Manages charge/discharge cycles, monitors health, and ensures active balancing to maximize lifespan.
- Thermal Management: Maintains optimal operating temperatures to enhance performance and safety.
- Fire Suppression System: Reduces fire risks, ensuring compliance with stringent safety standards.
- Cloud Connectivity: Allows users to remotely monitor and manage their system via apps and web platforms.
- Electrochemical Application: Many LFP batteries on the market today utilize CATL 280 Ah LFP cells, known for their stability, longevity, and high energy density.
Safety and Reliability
Safety concerns are top of mind with battery storage technologies, and many manufacturers have chosen to incorporate features like:
- Thermal Runaway Protection: Prevents overheating and related hazards.
- Flame-Retardant Materials: Minimizes fire risks.
- IP67 Protection: Ensures resistance to water and dust ingress.
- Grid Isolation and Low-Voltage Bus Architecture: Enhances user safety by minimizing electrical risks.
These measures reflect a commitment to creating reliable systems that prioritize user safety.
User Experience and Modularity
Modular designs are becoming more popular among battery manufacturers as their components reach mass market adoption. Modular systems allow for:
- Scalability: The ability to link multiple units supports small residential setups and larger commercial applications.
- User Interfaces: Cloud-based platforms and mobile apps enable real-time monitoring and control, empowering users to make informed decisions about their energy consumption.
Factors Affecting Solar Battery Lifespan
A solar battery’s lifespan is shaped by how it’s used, managed, and maintained, with LFP batteries offering unique resilience to these variables. This section unpacks the important factors influencing longevity, from depth of discharge to temperature, and explains why LFP often outlasts alternatives under the same conditions.
Backed by research like the Preger study and practical tips, we’ll show how these elements affect LFP’s performance in solar homes. Understanding these dynamics is key to getting the most out of your investment every year and cycle.
1. Depth of Discharge (DoD)
DoD refers to the percentage of a battery’s capacity able to be used before recharging.
- Impact: Deeper DoD accelerates wear and shortens battery life.
- Recommendations:
- Lead-acid: Operate at a 50% DoD to maximize lifespan.
- Thanks to their advanced chemistry, Lithium-ion can safely handle 80% DoD.
2. Temperature
Extreme temperatures can significantly degrade battery performance and lifespan.
- High Temperatures:
- Accelerates chemical reactions, leading to faster capacity degradation.
- Low Temperatures:
- Reduces capacity and efficiency, particularly in lead-acid batteries.
- Best Practices:
- Install batteries in temperature-controlled environments.
- Use insulation or cooling systems for protection.
3. Charging and Discharging Rates
Charging and discharging rates, measured by the C-rate (charge rate), can stress batteries and affect their lifespan.
- High C-Rates: Rapid charging or discharging generates heat, accelerating degradation.
- Optimal Practices:
- Use moderate C-rates as recommended by the manufacturer.
- Avoid frequent rapid charging unless necessary.
4. Maintenance
Proper maintenance can significantly extend a battery’s lifespan.
- Lead-Acid Maintenance:
- Regularly check electrolyte levels and add distilled water.
- Clean terminals to prevent corrosion.
- Lithium-Ion Maintenance:
- Minimal requirements; periodic monitoring for firmware updates and diagnostics suffices.
Insights from the Field
Our research tells one story, but the field tells more about battery longevity—and few know it better than Independent Energy of Hawaii, Oahu’s leading solar installer. Led by CEO Desta Rudolph, their team has managed thousands of solar energy system installations, offering a front-row view of how solar and battery storage systems perform in Hawaiian homes. These real-world insights highlight why Independent Energy’s work sets the standard for solar battery success.
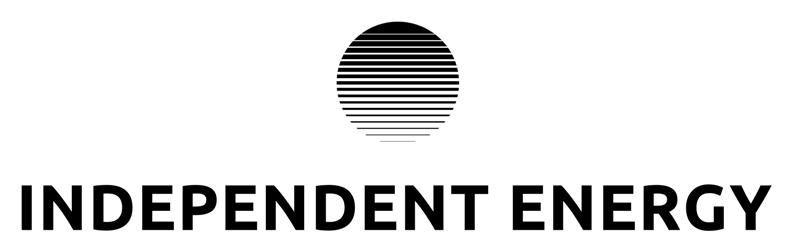
Warranty Claim Approvals vs. Denials
- Most warranty claims are approved, but the process is time-consuming and costly for installers.
- The biggest hurdle is that manufacturers require an on-site visit to obtain an RMA (Return Merchandise Authorization), meaning installers often have to make multiple trips—one to diagnose and get the RMA, and another to fix the issue.
- This increases labor costs for installers and causes delays and frustrations for customers.
- While claims generally get processed, the man-hours involved can make servicing BESS warranties a significant burden.
Conclusion
Solar batteries’ lifespans vary depending on their type, usage patterns, and environmental factors. While lead-acid batteries are more affordable upfront, lithium-ion batteries offer unparalleled longevity and reliability, especially those with LFP chemistry.
By considering your energy needs, maintaining optimal conditions, and choosing the right battery system, you can maximize the return on your investment. Whether for backup power or full off-grid independence, a well-maintained solar battery system is key to a sustainable energy future.